Fax #: 801-377-3697


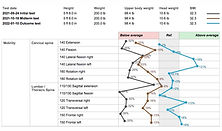
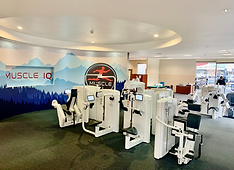
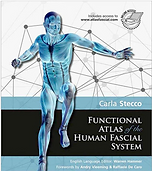
Journal of Muscle IQ
Journal of Muscle IQ - Volume 8
June of 2025
Unlocking Strength: Assessing Muscle Inhibition in Every Orthopedic Physical Therapy Patient
(Book Draft 10)
Prologue
​
After practicing physical therapy for 25 years, I wanted to put down on paper what I believe are some of the most important things I’ve learned for evaluating and treating patients. For example, one aspect that really interests me is helping patients return to active life by restoring strength. What is my focus for this book? First, the importance of finding weakness. Second, identifying the origin of that weakness. Third, eliminating as much of that weakness as possible.
Several years ago I started sharing my ideas on X. I learned quickly that all around the world every clinician has strong opinions. I learned that many will argue with you and say your ideas are wrong if your ideas are not backed up by scientific studies. Anecdotal evidence holds no weight when attempting to change the best clinical minds. This led me to search out and read all the latest studies about how muscle pain affects strength and I documented every aspect taught by each study about this subject. This book will include all the research I found on this subject, to back up what I have learned through my 25 years of treating my patients in an outpatient private practice setting.
During these last two years while writing this book, I’ve learned that the science is there, but the ideas I want to share in this book have not been organized in this way before. Each aspect has lots of science behind it, but no one has combined all the information together in this way before. And that is why I decided to write this book.
I hope to provide useful information that will be practical and applicable to the daily life of any outpatient, orthopedic physical therapist.
_______________________________________________________________________________________________________________
Chapter 1: Utilizing Light Touch to Assess Motor Cortex Inhibition in Muscle Weakness Associated with Pain
Introduction
​
Muscle weakness is a common clinical presentation in patients with musculoskeletal pain, often observed in conditions such as chronic low back pain (CLBP), rotator cuff tears (RCT), cervical radiculopathy, and medial collateral ligament tears (MCL). Emerging evidence suggests that this weakness is not solely due to peripheral muscle impairments but is significantly influenced by central nervous system mechanisms, particularly motor cortex inhibition. Pain-induced alterations in corticospinal excitability, characterized by increased short-interval intracortical inhibition (SICI) and reduced intracortical facilitation (ICF), can suppress motor output, leading to reduced muscle activation and force production. Recent studies highlight the potential of non-nociceptive somatosensory inputs, such as light touch, to modulate these inhibitory processes, offering a novel diagnostic tool for physical therapists to differentiate centrally mediated muscle weakness from peripheral causes.
This chapter explores the neurophysiological basis for using light touch over a painful body part to assess motor cortex inhibition as a contributor to muscle weakness. It provides a detailed, evidence-based protocol for physical therapists to implement this technique, supported by findings from 43 studies on pain, motor control, and somatosensory modulation. The chapter also discusses clinical implications, limitations, and future directions for integrating this approach into physical therapy practice.
Neurophysiological Basis for Light Touch Assessment
Pain and Motor Cortex Inhibition
​
Pain significantly alters motor cortex function, as demonstrated by transcranial magnetic stimulation (TMS) studies. In patients with chronic pain conditions, such as CLBP or RCT, motor-evoked potentials (MEPs) are reduced, indicating suppressed corticospinal excitability (Study 1, Study 9). This suppression is mediated by increased SICI, reflecting enhanced GABA_A receptor activity, and decreased ICF, linked to reduced glutamatergic facilitation (Study 4, Study 19). These changes are thought to be adaptive, restricting movement to protect the painful area from further injury (Study 19, Study 22). For example, a study on experimental muscle pain showed that pain increases SICI and reduces MEP amplitude, correlating with lower pain ratings when inhibition is stronger (Study 19). Similarly, in RCT patients, reduced MEP facilitation during voluntary deltoid activation suggests central reprogramming due to altered afferent input from the glenohumeral joint (Study 1).
This centrally mediated inhibition manifests clinically as muscle weakness, as seen in reduced peak torque in patients with musculoskeletal pain (Study 21). For instance, quadriceps strength in CLBP patients was significantly lower than in controls, with a lower central activation ratio indicating central, rather than peripheral, deficits (Study 22). These findings underscore that motor cortex inhibition is a key contributor to pain-related muscle weakness.
Role of Light Touch in Modulating Inhibition
Non-nociceptive somatosensory inputs, such as light touch, can modulate pain and motor cortex inhibition through sensory-motor integration. Tactile stimulation engages the primary somatosensory cortex (S1), which has strong monosynaptic connections to the primary motor cortex (M1) (Study 7). Studies demonstrate that homotopic tactile stimulation (e.g., to the painful area) triggers short-latency afferent inhibition (SAI), reducing corticospinal excitability, but also disinhibits local inhibitory circuits like SICI (Study 7, Study 8). This disinhibition can counteract pain-induced increases in SICI, potentially restoring motor output.
A key study on tactile-induced analgesia showed that light touch reduces pain perception at both spinal and cortical levels, with distinct spatiotemporal signatures in S1 (Study 23). Another study using a conditioning task found that tactile cues preceding painful stimuli diminish pain’s impact, suggesting competition between nociceptive and non-nociceptive inputs (Study 24). These findings indicate that light touch over a painful area may reduce motor cortex inhibition by modulating nociceptive drive and altering intracortical inhibitory networks, thereby facilitating muscle activation.
Clinical Rationale
The ability of light touch to modulate motor cortex inhibition provides a practical method for physical therapists to assess whether muscle weakness in a painful area is centrally mediated. By applying light touch and observing changes in muscle strength during the stimulation, therapists can infer the presence of motor cortex inhibition. An improvement in strength during tactile stimulation suggests that inhibition is reversible, pointing to a central mechanism, whereas no change may indicate peripheral or structural limitations. This approach leverages the neuroplasticity of sensory-motor pathways and aligns with evidence that somatosensory inputs can enhance corticomotor excitability (Study 5, Study 8).
​
Protocol for Assessing Motor Cortex Inhibition with Light Touch
​
Objective
To determine if muscle weakness in a painful body part is caused by motor cortex inhibition using light touch as a diagnostic tool.
Equipment
-
Dynamometer (handheld or isokinetic, e.g., Cybex) or manual muscle testing (MMT) equipment for strength assessment.
Patient Selection
-
Inclusion Criteria: Patients with musculoskeletal pain (e.g., CLBP, RCT, neck pain, knee pain) for >6 months, exhibiting muscle weakness in the painful area, and who have undergone conservative treatment (e.g., anti-inflammatory medication, physical therapy) (Study 1, Study 36).
-
Exclusion Criteria: Patients with neurological disorders (e.g., stroke, Parkinson’s), acute injuries (<6 months), or contraindications to strength testing (e.g., recent surgery, severe joint instability).
Procedure
-
Patient Preparation:
-
Explain the procedure to the patient, emphasizing that light touch is non-invasive and aims to assess the cause of muscle weakness.
-
Position the patient comfortably to access the painful body part (e.g., seated for shoulder, supine for low back).
-
Clean the skin over the painful area with an alcohol wipe to ensure consistent tactile stimulation.
-
-
Baseline Strength Assessment:
-
Select a target muscle associated with the painful area (e.g., deltoid for RCT, quadriceps for knee pain, lumbar multifidus for CLBP).
-
Measure baseline muscle strength using a dynamometer for objective torque output (e.g., peak torque in Nm/kg) or MMT (0–5 scale) for clinical settings (Study 21, Study 22).
-
Perform three trials, allowing 30 seconds rest between trials, and record the average strength value.
-
Note the patient’s pain level (0–10 numeric pain rating scale) during testing to account for pain interference.
-
-
Light Touch Application:
-
Identify the painful area (e.g., over the rotator cuff for RCT, L4–L5 region for CLBP).
-
Using the middle three fingers (index, middle, ring) of the dominant hand, apply light, stationary touch to the painful area with gentle pressure (sufficient to indent the skin slightly but not cause discomfort). Maintain contact for 5 seconds. Ensure the touch is homotopic (directly over the painful site) to maximize S1-M1 interaction (Study 6, Study 7).
-
Monitor the patient to ensure the touch remains non-painful; adjust pressure if discomfort is reported.
-
-
Strength Assessment During Touch:
-
While maintaining the light touch (fingers stationary on the painful area), instruct the patient to perform the strength test for the target muscle (e.g., shoulder abduction for deltoid, knee extension for quadriceps).
-
Measure strength using the same method (dynamometer or MMT) as in the baseline, performing three trials during the 5-second touch period. If using a dynamometer, ensure rapid trials to fit within the timeframe; for MMT, assess resistance against manual pressure.
-
Record the average strength value and note any changes in pain level during testing.
-
Allow a 2-minute rest period, then repeat the light touch and strength assessment to confirm consistency of findings.
-
-
Control Condition (Optional):
-
To enhance diagnostic specificity, apply light touch with the middle three fingers to a heterotopic (non-painful) area (e.g., contralateral limb or adjacent non-painful region) for 5 seconds and repeat strength testing during the touch. Heterotopic stimulation is less likely to modulate pain-specific inhibition (Study 6).
-
Compare strength changes between homotopic and heterotopic conditions.
-
Interpretation of Results
• Positive Response (Central Inhibition Likely): An increase in muscle strength (e.g., higher torque or MMT score) during homotopic light touch suggests that motor cortex inhibition is contributing to weakness. This aligns with studies showing that tactile stimulation reduces SICI and enhances corticomotor excitability (Study 5, Study 8).
• No Change (Peripheral or Mixed Cause): If strength remains unchanged during light touch, weakness may be due to peripheral factors (e.g., muscle atrophy, structural damage) or irreversible central changes. Further diagnostic tests (e.g., EMG, imaging) are warranted.
• Pain Modulation: A reduction in pain rating during touch supports the involvement of tactile-induced analgesia, reinforcing the role of sensory-motor integration (Study 23).
Recording and Documentation
• Document baseline and during-touch strength values, pain ratings, and patient feedback.
• Note the specific muscle tested, touch application site, and any differences between homotopic and heterotopic conditions.
This light touch protocol offers a non-invasive, cost-effective method for physical therapists to assess the central contribution to muscle weakness in painful conditions. Identifying motor cortex inhibition as a cause can guide targeted interventions, such as sensory-motor training, aerobic exercise, or manual therapy, which have been shown to enhance corticomotor excitability and reduce inhibition (Study 5, Study 36, Study 39). For example, moderate-intensity aerobic exercise decreases SICI and improves motor learning, which could complement tactile interventions (Study 5). Additionally, this approach may inform rehabilitation by prioritizing strategies that address neuroplastic changes over purely strength-based exercises, which may be less effective in the presence of inhibition (Study 1).
Limitations and Considerations
• Variability in Response: Patient-specific factors, such as pain chronicity, psychological distress, or cortical reorganization, may influence the effectiveness of light touch (Study 1, Study 22). For instance, CLBP patients with high psychological distress showed lower central activation ratios (Study 22).
• Diagnostic Specificity: While light touch can indicate central inhibition, it does not rule out concurrent peripheral contributions. Complementary assessments (e.g., ultrasound, EMG) are recommended.
• Standardization: The pressure applied by the fingers must be consistent to ensure reliable results. Therapist training is essential to minimize variability.
• Contraindications: Avoid light touch in areas with hypersensitivity (e.g., allodynia) or acute inflammation, as it may exacerbate pain.
• Timing Constraints: Strength testing during the 5-second touch period requires rapid execution, which may be challenging for some dynamometer-based assessments.
Future Directions
Further research is needed to validate the stationary light touch protocol across diverse pain conditions and establish normative strength change thresholds for clinical significance. Integrating TMS or electroencephalography (EEG) with this protocol could provide direct measures of cortical excitability changes, enhancing diagnostic precision. Additionally, longitudinal studies should explore whether repeated light touch interventions can sustain reductions in motor cortex inhibition, potentially serving as a therapeutic tool. Investigating the role of subcortical regions in tactile modulation, as suggested by one study (Study 6), may also deepen our understanding of the underlying mechanisms.
Conclusion
Light touch over a painful body part, applied using the tester’s middle three fingers for 5 seconds, offers a promising diagnostic tool for physical therapists to assess motor cortex inhibition as a contributor to muscle weakness in musculoskeletal pain. By leveraging the modulatory effects of non-nociceptive somatosensory inputs on sensory-motor pathways, this simplified technique provides insights into central mechanisms of weakness, guiding personalized rehabilitation strategies. The protocol outlined in this chapter, grounded in robust neurophysiological evidence, is practical for clinical settings and aligns with the evolving understanding of pain’s impact on motor control. As research advances, this approach may become a cornerstone of physical therapy diagnostics, enhancing outcomes for patients with pain-related muscle dysfunction.
References
• Study 1: Chronic rotator cuff tear patients and motor cortex changes. Central neuromuscular dysfunction of the deltoid muscle in patients with chronic rotator cuff tears. https://jorthoptraumatol.springeropen.com/articles/10.1007/s10195-009-0061-7
• Study 4: Muscle pain and differential modulation of SICI and ICF. Muscle pain differentially modulates short interval intracortical inhibition and intracortical facilitation in primary motor cortex. https://www.jpain.org/article/S1526-5900(11)00870-4/fulltext
• Study 5: Aerobic exercise effects on motor learning and cortical excitability. Acute Aerobic Exercise at Different Intensities Modulates Motor Learning Performance and Cortical Excitability in Sedentary Individuals. https://www.eneuro.org/content/10/11/ENEURO.0182-23.2023
• Study 6: Tactile stimulation and afferent inhibition in M1. Modulation of Motor Cortical Inhibition and Facilitation by Touch Sensation from the Glabrous Skin of the Human Hand. https://www.eneuro.org/content/11/3/ENEURO.0410-23.2024
• Study 7: S1-M1 connections and motor control. The influence of sensory afferent input on local motor cortical excitatory circuitry in humans. https://www.ncbi.nlm.nih.gov/pmc/articles/PMC4386965/
• Study 8: Concussion, exercise, and cortical plasticity. Effects of Acute Aerobic Exercise on Motor Cortex Plasticity in Individuals With a Concussion History. https://uwspace.uwaterloo.ca/bitstream/handle/10012/18930/Khan_Madison.pdf?sequence=5&isAllowed=y
• Study 19: Pain-induced changes in MEP and TEP N45 peak. Alterations in cortical excitability during pain: A combined TMS-EEG Study. https://www.biorxiv.org/content/10.1101/2023.04.20.537735v2
• Study 21: Experimental muscle pain and central force inhibition. Inhibition of maximal voluntary contraction force by experimental muscle pain: A centrally mediated mechanism. https://onlinelibrary.wiley.com/doi/10.1002/mus.10225
• Study 22: Quadriceps strength and central activation in CLBP. Pain-Related Factors Contributing to Muscle Inhibition in Patients With Chronic Low Back Pain. https://journals.lww.com/clinicalpain/fulltext/2005/05000/pain_related_factors_contributing_to_muscle.6.aspx
• Study 23: Tactile-induced analgesia at spinal and cortical levels. A spatiotemporal signature of cortical pain relief by tactile stimulation: An MEG study. https://www.sciencedirect.com/science/article/abs/pii/S1053811916000951
• Study 24: Pain-tactile interaction in conditioning tasks. When touch predicts pain: predictive tactile cues modulate perceived intensity of painful stimulation independent of expectancy. https://www.degruyter.com/document/doi/10.1016/j.sjpain.2015.09.007/html
• Study 36: Strength training in CLBP. Effectiveness of a Group-Based Progressive Strength Training in Primary Care to Improve the Recurrence of Low Back Pain Exacerbations and Function: A Randomised Trial. https://www.mdpi.com/1660-4601/17/22/8326
• Study 39: Combined physiotherapy for shoulder pain. Which Multimodal Physiotherapy Treatment Is the Most Effective in People with Shoulder Pain? A Systematic Review and Meta-Analyses. https://mdpi-res.com/d_attachment/healthcare/healthcare-12-01234/article_deploy/healthcare-12-01234.pdf?version=1718886349
___________________________________________________________________________
Chapter 2: The Neurophysiological Impact of Muscle Pain on the Motor Cortex
Introduction to Muscle Pain and Motor Function (for better patient outcomes)
To understand how muscle inhibition affects every orthopedic physical therapy patient we first must cover some background. Muscle pain influences motor function, resulting in muscle weakness, and a decreased ability for the patient to perform important daily tasks. This weakening of the muscles stems from complex interactions between peripheral nociceptive inputs and the central nervous system, particularly the Motor Cortex—the brain region responsible for initiating and coordinating voluntary movements. Understanding these anatomical and physiological processes can help us improve patient outcomes.
This section explores how afferent inputs from painful muscle tissue and fascia reach the brain and alter Motor Cortex activity, leading to muscle weakness.
(Knowing how this works can also help us understand how to restore full Motor Cortex activity and muscle strength).
Anatomy of the Pain Pathway and Motor Cortex
​
To grasp how muscle pain affects motor function, we must first examine the relevant anatomical structures and their roles.
-
Peripheral Nociceptors and Afferent Fibers
-
Muscle pain originates from nociceptors—specialized sensory receptors embedded in skeletal muscle tissue and fascia. These receptors detect noxious stimuli such as mechanical damage, chemical irritants (e.g., lactic acid), or inflammation.
-
Two primary types of afferent fibers carry pain signals from muscles to the spinal cord:
-
Group III (Aδ) fibers: Thin, myelinated fibers that transmit sharp, localized pain rapidly (conduction velocity ~2.5–30 m/s).
-
Group IV (C) fibers: Unmyelinated fibers that conduct dull, diffuse pain more slowly (conduction velocity ~0.5–2 m/s).
-
-
These fibers synapse in the dorsal horn of the spinal cord, a critical relay station for sensory processing.
-
-
Spinal Cord Integration
-
In the dorsal horn, nociceptive signals are processed by second-order neurons in laminae I, II, and V. These neurons integrate inputs from muscle nociceptors with other sensory information, such as touch or pressure, before relaying the signal upward. (Integration of Touch and Pain signals can decrease the effect of the pain signal before reaching the Motor Cortex).
-
The spinothalamic tract, a major ascending pathway, carries these pain signals from the spinal cord to the brainstem and thalamus. This tract crosses to the contralateral side of the body, ensuring that pain from the right arm, for example, is processed by the left brain hemisphere.
-
The motor cortex does not differentiate between pain originating from fascia and pain from muscle tissue. Afferent fibers from both muscle and fascia converge and synapse within the same region of the spinal cord’s dorsal horn, where they are integrated and relayed via shared secondary neurons along the spinothalamic tract to higher brain centers. This neuroanatomical convergence supports the indistinguishable processing of nociceptive signals from these tissues (Mense, 2019).
-
Source: Mense, S. (2019). Innervation of the thoracolumbar fascia. European Journal of Pain Supplements, 23(1), 7–14. https://doi.org/10.1002/ejp.1284
-
-
Thalamus and Cortical Projections
-
The thalamus acts as a sensory relay hub, receiving spinothalamic inputs and distributing them to various cortical regions. For pain, the ventral posterior lateral (VPL) nucleus of the thalamus is particularly relevant, projecting to both the sensory and motor cortices.
-
Pain signals reach two key cortical areas:
-
Primary Somatosensory Cortex (S1): Located in the postcentral gyrus, S1 processes the sensory aspects of pain, such as its location and intensity.
-
Primary Motor Cortex (M1): Situated in the precentral gyrus, M1 generates motor commands for voluntary movement. Its involvement in pain processing reflects the tight linkage between sensory input and motor output.
-
-
-
Motor Cortex Anatomy
-
The Motor Cortex (M1) contains a topographic map of the body, known as the motor homunculus, where specific regions control specific muscle groups (e.g., the hand area is lateral, the leg area is medial).
-
Rewritten Paragraph:
-
Recent research has revealed that the cortical homunculus map exhibits partial bilateral representation, with each cerebral hemisphere primarily controlling and processing sensory and motor functions for the contralateral side of the body, but also containing smaller regions dedicated to the ipsilateral side. Specifically, while the primary motor and sensory cortices predominantly map to the opposite side of the body, there is evidence of limited ipsilateral representation, particularly in areas such as the premotor and parietal regions, which contribute to coordinated movements and sensory integration for both sides of the body (Merrick et al., 2022).
-
Source: Merrick, C., Merrill, B., Velez, J., Bundy, D. T., Leuthardt, E. C., & Downey, J. E. (2022). Left hemisphere dominance for bilateral kinematic encoding in the human brain. eLife, 11, e69976. https://doi.org/10.7554/eLife.69976
-
Pyramidal cells in layer V of M1 are the primary output neurons, sending axons via the corticospinal tract to synapse with motor neurons in the spinal cord’s ventral horn. These neurons drive muscle contraction.
-
Physiology of Pain-Induced Motor Cortex Inhibition
​
When painful stimuli from muscle tissue or fascia activate nociceptors, the resulting afferent inputs trigger a cascade of physiological changes that ultimately suppress Motor Cortex activity. This inhibition underlies the muscle weakness observed in most patients presenting to the clinic with painful conditions.
-
Nociceptive Signal Transmission
-
Upon activation, muscle and fascia nociceptors release neurotransmitters like glutamate and substance P at their spinal cord synapses. These chemicals excite second-order neurons, amplifying the pain signal.
-
The signal ascends via the spinothalamic tract to the thalamus, which relays it to the Motor Cortex. Unlike touch or proprioception, which primarily target S1, nociceptive inputs influence M1 directly and indirectly through thalamo-cortical connections.
-
-
Motor Cortex Response to Pain
-
Studies using transcranial magnetic stimulation (TMS) demonstrate that acute muscle pain reduces Motor Cortex excitability. TMS applies magnetic pulses to stimulate M1, eliciting motor evoked potentials (MEPs)—electrical responses in target muscles that reflect cortical output strength.
-
In experimental models, injecting hypertonic saline (5%) into muscles mimics musculoskeletal pain and consistently decreases MEP amplitude. This reduction occurs without changes to the H-reflex, a spinal-level reflex, indicating that the inhibition is cortical, not spinal.
-
Capsaicin, a chemical irritant applied to skin or muscle, produces similar results, further confirming that pain suppresses M1 activity.
-
-
Mechanisms of Inhibition
-
Several mechanisms may explain this cortical inhibition:
-
GABAergic Inhibition: Pain signals may activate inhibitory interneurons within M1 that release gamma-aminobutyric acid (GABA). GABA hyperpolarizes pyramidal cells, reducing their firing rate and thus weakening motor output.
-
Thalamo-Cortical Modulation: The thalamus, influenced by pain inputs, may alter its excitatory drive to M1, decreasing glutamate release and dampening cortical activity.
-
Sensory-Motor Integration: Painful inputs to S1 may indirectly inhibit M1 via cortico-cortical connections. For instance, increased S1 activation during pain (seen in fMRI studies) could trigger a feedback loop that suppresses M1 excitability.
-
-
The net result is fewer or weaker action potentials traveling down the corticospinal tract, leading to reduced motor neuron activation and weaker muscle contractions.
-
-
Physiological Evidence
-
A systematic review of TMS and EEG studies found moderate to strong evidence of reduced corticomotor excitability during and after acute muscle pain. This effect is consistent across pain induction methods (e.g., saline, capsaicin), though its intensity varies with pain duration and severity.
-
Chronic pain, such as in low back pain, shows similar hypoexcitability, with decreased levels of N-Acetylaspartate (a neuronal health marker) in M1, suggesting potential long-term changes.
-
Clinical Implications for Physical Therapists
The inhibition of the Motor Cortex by muscle pain has direct relevance to physical therapy:
-
Muscle Weakness: Reduced M1 excitability explains why patients with muscle pain often exhibit weakness without actual structural damage. For example, a patient with a painful knee may struggle to extend their knee against manual resistence even if muscle fibers are not torn.
-
Therapeutic Strategies: Identify all of the muscles that are weak. If there is pain, you will find weakness. Remember that weakness does not mean torn muscles, it may be muscle inhibition from the Motor Cortex.
Conclusion
When afferent inputs from painful muscle tissue reach the brain, they inhibit the Motor Cortex through a combination of direct nociceptive effects and sensory-motor interactions. This process, rooted in the anatomy of nociceptive pathways and the physiology of cortical inhibition, results in reduced motor output and muscle weakness. For physical therapists, recognizing these mechanisms provides a foundation for designing interventions that address both pain and its motor consequences, ultimately improving patient outcomes.
​
___________________________________________________________________________
Chapter 3: Assessing Motor Cortex Inhibition in Muscle Pain through Light Touch
​
Introduction
Muscle pain often results in weakness, partly due to inhibition of the Motor Cortex triggered by nociceptive inputs. While this cortical suppression diminishes motor output, its specific contribution to weakness can be challenging to isolate clinically. This section will introduce how light touch can be used as an assessment tool to detect the presence of Motor Cortex inhibition. By leveraging the physiological interaction between light touch signals and pain signals, physical therapists can assess the cortical component of weakness, improving diagnostic accuracy and treatment planning.
The Dorsal Horn’s Role in Sensory-Motor Dynamics
The dorsal horn, located in the posterior gray matter of the spinal cord, integrates sensory inputs that influence motor responses. Organized into laminae I–VI, it processes nociceptive signals from painful muscles via Group III (Aδ) and Group IV (C) fibers, which synapse in laminae I, II, and V, releasing glutamate and substance P to excite second-order neurons. These signals ascend through the spinothalamic tract to the Motor Cortex, where they inhibit pyramidal cell activity, reducing corticospinal output and contributing to muscle weakness. Simultaneously, touch signals from Aβ fibers, entering laminae III–VI, in the dorsal horn. This overlap and integration at the dorsal horn allows touch to modify the nociceptive input affecting the Motor Cortex, offering insight into how much of the muscle weakness is due to Motor Cortex inhibition.
Physiological Modulation of Nociceptive Signals
Nociceptive signals from a painful muscle excite second-order neurons in the dorsal horn, enhancing inhibition in the Motor Cortex and decreasing motor evoked potentials (MEPs), which weakens muscle contraction. However, touch via Aβ fibers activates inhibitory interneurons that release GABA or glycine, hyperpolarizing these second-order neurons and reducing their firing rate. This suppression diminishes the nociceptive signal relayed to the Motor Cortex, lessening its inhibitory effect and allowing greater corticospinal activation. Therapists can use this response to evaluate the cortical contribution to weakness: a notable strength increase with touch indicates significant Motor Cortex inhibition, while minimal change suggests other factors, such as local muscle pathology, other neurological pathology, may be present.
Light Touch as an Assessment Method
Applying light touch at or near the paraspinal muscles at the same spinal level as the painful muscle’s myotome enhances this diagnostic approach due to segmental alignment. The paraspinals (innervated by posterior rami) and limb muscles (innervated by anterior rami) share spinal segments (e.g., L2–L4 for quadriceps). For a patient with quadriceps pain, touch at the L2–L4 paraspinals activates Aβ fibers, triggering inhibitory interneurons that reduce dorsal horn nociceptive output and, consequently, Motor Cortex suppression. A therapist can then assess strength—manually or with dynamometry—before and during touch. For example, if quadriceps strength improves significantly with light touch at or near L3, it suggests that Motor Cortex inhibition, driven by pain, is a major factor in the weakness. Little improvement, however, could point to structural or other neurological causes.
Clinical Application and Insights
This technique provides physical therapists with a practical method to quantify Motor Cortex inhibition’s role in muscle weakness. By comparing baseline strength (during pain) to strength with light touch, therapists can estimate the cortical component. TMS studies support this, demonstrating increased MEP amplitude when nociceptive input decreases, linking dorsal horn modulation to cortical recovery.
Conclusion
Strategic light touch at the painful muscle, or at the paraspinal muscles, aligned with the painful muscle’s spinal level, modifies dorsal horn activity to uncover the degree of Motor Cortex inhibition in muscle weakness. By activating inhibitory interneurons to reduce nociceptive signals, this method allows therapists to assess how much cortical suppression contributes to a patient’s motor deficit. This anatomically precise technique equips physical therapists with a clearer understanding of pain-related weakness, enabling them to design rehabilitation strategies that effectively target both cortical and peripheral factors.
_____________________________________________________________
Chapter 4: “Global Muscle Output Reduction Triggered by Active Use of Painful or Weak Muscles”
Key Points
-
Research suggests that actively using a painful or weak muscle can cause a global decrease in muscle output, affecting muscles beyond the painful area.
-
It seems likely that this occurs through central nervous system inhibition, reducing the ability to fully activate muscles across the body.
-
The evidence leans toward pain sometimes causing widespread muscle inhibition, demonstrated acutely, and in chronic conditions like low back pain and fibromyalgia, but the exact mechanisms may vary.
Overview
When you actively use a painful or weak muscle, studies show it can lead to weaker muscle contractions not just in that muscle, but in others too. This happens because pain signals can mess with how your brain and spinal cord control muscles, making it harder to use them strongly everywhere.
How It Works
Research, like a study from 2002, found that if you have muscle pain, the force you can generate with other muscles drops, and this is due to your central nervous system, not the muscles themselves (Inhibition of maximal voluntary contraction force by experimental muscle pain: a centrally mediated mechanism). Another study from 2005 on chronic low back pain showed that patients had weaker quadriceps, even though the pain was in their back, suggesting pain can spread its effect (Pain-related factors contributing to muscle inhibition in patients with chronic low back pain: an experimental investigation based on superimposed).
Comprehensive Analysis of Global Muscle Output Decrease Due to Active Use of Painful or Weak Muscles
This section provides a detailed examination of how actively using a painful or weak muscle triggers a global decrease in muscle output, focusing on the central nervous system mechanisms involved. The analysis is grounded in recent neuroscience and rehabilitation research.
Introduction
Muscle pain, whether acute or chronic, is known to impair motor function, often manifesting as weakness in the affected muscle. However, research has demonstrated that actively using a painful or weak muscle can extend this inhibition beyond the local site, leading to a global decrease in muscle output. This phenomenon is evident in acute pain and conditions like chronic low back pain and fibromyalgia, where pain-related factors contribute to widespread motor deficits. This analysis compiles factual information from experimental and clinical studies to elucidate these effects.
Central Mediation of Muscle Inhibition
Graven-Nielsen et al. (2002) conducted a study titled “Inhibition of maximal voluntary contraction force by experimental muscle pain: a centrally mediated mechanism” published in Muscle & Nerve (Inhibition of maximal voluntary contraction force by experimental muscle pain: a centrally mediated mechanism). The researchers induced experimental muscle pain and measured its effect on maximal voluntary contraction (MVC) torque and peripheral contractile properties. They found that muscle pain significantly reduced the torque produced by isometric knee extension, with a mean reduction observed, but the contractile properties assessed by twitch interpolation were not affected. This indicates that the force inhibition is centrally mediated, as the peripheral muscle properties, such as twitch torque, remained unchanged, suggesting that the weakness is due to central processes rather than peripheral damage.
Pain-Related Factors in Chronic Low Back Pain
Verbunt et al. (2005) in their study “Pain-related factors contributing to muscle inhibition in patients with chronic low back pain: an experimental investigation based on superimposed” published in The Clinical Journal of Pain (Pain-related factors contributing to muscle inhibition in patients with chronic low back pain: an experimental investigation based on superimposed) evaluated muscle strength and central activation ratio in patients with chronic low back pain compared to healthy controls. They measured peak torque of the quadriceps muscle using a Cybex dynamometer and applied superimposed electrical stimulation to assess submaximal performance. The results showed that patients with chronic low back pain had significantly lower peak torque (mean 1.95 Nm/kg vs. 3.16 Nm/kg in controls, P < 0.01) and lower central activation ratios (P < 0.05). This indicates that pain-related factors, including psychological distress and current pain levels, contribute to muscle inhibition, affecting muscles distant from the pain site, such as the quadriceps, when actively used.
Global Measures of Strength in Musculoskeletal Pain
A systematic review and meta-analysis by Verdini et al. (2023) titled “Do people with musculoskeletal pain differ from healthy cohorts in terms of global measures of strength? A systematic review and meta-analysis” published in Clinical Rehabilitation (Do people with musculoskeletal pain differ from healthy cohorts in terms of global measures of strength? A systematic review and meta-analysis) investigated whether individuals with musculoskeletal pain exhibit different multi-joint strength capacities compared to healthy cohorts. The review included studies on various conditions such as knee osteoarthritis, fibromyalgia, chronic low back pain, and rheumatoid arthritis. For fibromyalgia, the meta-analysis showed reduced chest press and leg press strength in adult women with the condition compared to active community women, with standardized mean differences (Hedge’s g) of -0.34 (95% CI [-0.64, -0.03]) for chest press and -0.25 (95% CI [-0.49, -0.02]) for leg press. This indicates that musculoskeletal pain, particularly in conditions like fibromyalgia, leads to a widespread decrease in muscle strength affecting both upper and lower limbs, suggesting that active use of painful muscles exacerbates global weakness.
Impact on Muscle Strength and Activity
Graven-Nielsen and Arendt-Nielsen (2008) in their review “Impact of clinical and experimental pain on muscle strength and activity” published in Current Rheumatology Reports (Impact of clinical and experimental pain on muscle strength and activity) discussed how muscle pain affects motor control. They noted that muscle pain reduces maximal voluntary contraction and endurance time during submaximal contractions, with specific findings showing no increase in electromyographic activity at rest but significant reductions in MVC and endurance. The review also highlighted that muscle pain causes adaptive changes in coordination during dynamic exercises, with increased muscle activity reflecting reorganized muscle coordination and strategy. This supports an adaptive model where reduced agonistic muscle activity is eventually advanced by changed antagonistic muscle activity, indicating that active use of a painful muscle leads to global motor deficits [4].
Summary Table of Key Findings
Aspect
Finding
Evidence Source
Central Mediation
Muscle pain inhibits maximal voluntary contraction force through central mechanisms, not peripheral damage
Graven-Nielsen et al. (2002) [1] (Inhibition of maximal voluntary contraction force by experimental muscle pain: a centrally mediated mechanism)
Chronic Low Back Pain
Patients show decreased quadriceps strength and lower central activation ratios, linked to pain and psychologic distress
Verbunt et al. (2005) [2] (Pain-related factors contributing to muscle inhibition in patients with chronic low back pain: an experimental investigation based on superimposed)
Fibromyalgia and Multi-Joint Strength
Adult women with fibromyalgia exhibit reduced chest press and leg press strength compared to controls
Verdini et al. (2023) [3] (Do people with musculoskeletal pain differ from healthy cohorts in terms of global measures of strength? A systematic review and meta-analysis)
Pain’s Effect on Coordination
Muscle pain reduces maximal voluntary contraction, endurance, and alters coordination, supporting adaptive model
Graven-Nielsen et al. (2008) [4] (Impact of clinical and experimental pain on muscle strength and activity)
This table summarizes the key effects, providing a structured overview of the research findings.
Conclusion
In conclusion, the active use of a painful or weak muscle triggers a global decrease in muscle output through centrally mediated mechanisms. Studies have consistently shown that pain inhibits maximal voluntary contraction force, reduces muscle strength in distant muscles, and alters muscle coordination and activity. These effects are observed in both experimental pain models and clinical conditions such as chronic low back pain and fibromyalgia, highlighting the widespread impact of muscle pain on motor function.
Key Citations
________________________________________________________________________
Chapter 5: Unlocking Strength - Integrating Pain and Motor Insights for Tailored Physical Therapy
​
Introduction
​
Muscle pain and weakness present complex challenges for physical therapists, intertwining local motor deficits with broader systemic effects. Drawing from the insights of the previous chapters—Motor Cortex inhibition from pain, dorsal horn modulation via touch, and global muscle output reduction—this chapter synthesizes these findings into a practical framework. By evaluating patients systematically, physical therapists can identify weak muscles, assess the scope of inhibition, and pinpoint spinal segments for targeted treatment, optimizing exercises and interventions for each individual’s condition.
​
Evaluating Muscle Weakness
​
Physical therapists begin by assessing patients to locate weak muscles, using tools like manual muscle testing or dynamometry. Chapter 1 highlighted how pain inhibits the Motor Cortex, reducing corticospinal output and causing weakness in the affected muscle. For instance, a patient with a painful knee may exhibit reduced knee extension strength. This initial evaluation establishes a baseline, identifying the primary site of motor dysfunction.
​
Testing for Global Weakness
​
Next, therapists ask the patient to actively use the identified weak muscle—such as performing a seated knee extension —and then test the strength of an unrelated muscle, like the deltoid. Chapter 3 demonstrated that active use of a painful or weak muscle triggers a global decrease in muscle output, where distant muscles weaken due to central inhibition. If the deltoid weakens after quadriceps activation, it confirms a systemic effect, suggesting that pain-driven neural mechanisms extend beyond the local site.
​
Palpation to Isolate Spinal Influence
​
To refine the approach, therapists test the newly weakened muscle (e.g., deltoid) during seated knee extension, while simultaneously palpating the deep paraspinal muscles at the spinal level corresponding to the original weak muscle’s myotome (e.g., L2–L4 for quadriceps). Chapter 2 showed that touch at the paraspinal region activates Aβ fibers, reducing nociceptive signals in the dorsal horn and lessening Motor Cortex inhibition. If deltoid strength suddenly returns to normal during light touch at L3, it indicates that spinal-level modulation at that segment can eliminate the global weakening. This points to a key treatment locus, linking the original weakness and its systemic impact to a specific spinal level.
​
Tailoring Treatment and Exercises
​
These findings guide individualized interventions. When palpation at a spinal segment (e.g., L3) reverses global weakening, treatment should focus there—potentially through manual therapy, targeted stretching, or exercises like paraspinal strengthening—to reduce nociceptive input and enhance Motor Cortex excitability. For the original weak muscle (e.g., quadriceps), therapists can design progressive resistance exercises, starting with low intensity to avoid exacerbating global inhibition, then scaling up as spinal modulation takes effect. If global weakening persists despite palpation, therapists might consider broader central nervous system strategies, such as graded motor imagery, to address widespread inhibition.
​
Conclusion
​
By integrating knowledge of Motor Cortex inhibition, dorsal horn modulation, and global muscle output reduction, physical therapists can craft precise, patient-specific plans. Evaluating weak muscles, testing for systemic effects during active use, and using paraspinal palpation to identify critical spinal segments allow therapists to pinpoint where treatment will most effectively restore strength. This approach not only addresses the original weakness but also mitigates its global consequences, empowering patients with tailored exercises and interventions to reclaim their motor function.
​
__________________________________________________________________________
Chapter 6: Final Thoughts
​
The ability to assess muscle inhibition will help every orthopedic physical therapist treat their patient. It should be understood…
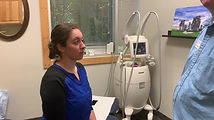
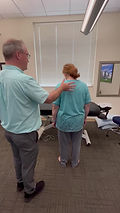
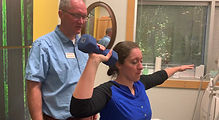
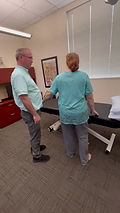



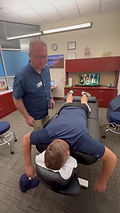